The problems in this masterclass package are intended to offer students the opportunity to engage in a key mathematical activity: moving from particular instances to general cases. Along the way, students can notice patterns, make conjectures and choose representations to help justify and prove.
Summing Consecutive Numbers
15 = 7 + 8 and 10 = 1 + 2 + 3 + 4. Can you say which numbers can be expressed as the sum of two or more consecutive integers?
Problem
Summing Consecutive Numbers printable worksheet
Watch the video below to see how numbers can be expressed as sums of consecutive numbers.
Investigate the questions posed in the video and any other questions you come up with.
Can you draw any conclusions?
Can you support your conclusions with convincing arguments or proofs?
If you are unable to view the video, click below to reveal an alternative version of the problem.
Charlie has been thinking about sums of consecutive numbers. Here is part of his working out:

Alison looked over Charlie's shoulder:
"I wonder if we could write every number as the sum of consecutive numbers?"
"Some numbers can be written in more than one way! I wonder which ones?"
"$9$, $12$ and $15$ can all be written using three consecutive numbers. I wonder if all multiples of $3$ can be written in this way?"
"Maybe you could write the multiples of $4$ if you used four consecutive numbers..."
Choose some of the questions above, or pose some questions of your own, and try to answer them.
Can you support your conclusions with convincing arguments or proofs?
Getting Started
Start by trying some simple cases; perhaps start by exploring what happens when you add two or three consecutive numbers.
$1 + 2 + 3 = 6$
$2 + 3 + 4 = 9$
Can you explain why the total has gone up by $3$?
Student Solutions
Swaathi, from Garden International School, started by listing the numbers up to 15 and trying to represent them as sums of consecutive numbers:
2
3 = 1+2
4
5 = 2+3
6 = 1+2+3
7 = 3+4
8
9 = 4+5 = 2+3+4
10 = 1+2+3+4
11 = 5+6
12 = 3+4+5
13 = 6+7
14 = 2+3+4+5
15 = 7+8 = 4+5+6 = 1+2+3+4+5
We can't write every number as a sum of consecutive numbers - for example, 2, 4 and 8 can't be written as sums of consecutive numbers. In the above, 9 and 15 were the only numbers that I could find that could be written in more than one way.
Many people spotted the pattern that all odd numbers (except 1) could be written as the sum of two consecutive numbers. For example, Matilda and Tamaris wrote:
If you add two consecutive numbers together, the sum is an odd number, e.g.
1+2=3
2+3=5
3+4=7
4+5=9
5+6=11
6+7=13
and so on...
Well done to pupils from Kenmont Primary School who noticed this, and explained that an Odd plus an Even is always Odd.
Some spotted a similar pattern for multiples of 3. Julia and Lizzie said:
If you add any 3 consecutive numbers together it will always equal a multiple of 3, e.g.
1+2+3=6
2+3+4=9
3+4+5=12
4+5+6=15
5+6+7=18
Continuing with the patterns, the Lumen Christi grade 5/6 maths extension program team sent us:
We discovered that the sum of four consecutive numbers gave us the number sequence 10, 14, 18, 22, 26, 30, and so on. They were all even numbers that had an odd number as half of its total.
1+2+3+4=10
2+3+4+5=14
3+4+5+6=18...
Heather from Wallington High School for Girls explained this pattern:
10 - 1+2+3+4
14 - 2+3+4+5
18 - 3+4+5+6
22 - 4+5+6+7
In all the columns, each place adds 1 each time, so in total you add 4 each time.
Ruby said:
Numbers which are multiples of 5, starting with 15, are sums of 5 consecutive numbers:
1+2+3+4+5=15
2+3+4+5+6=20
3+4+5+6+7=25...
Fergus and Sami noticed a similar pattern:
If you allow negative numbers, you can find a sum for any multiple of 7 easily. Each time you add one number either side of the sum, your sum increases by 7, e.g.
3+4=7
2+3+4+5=14
1+2+3+4+5+6=21
0+1+2+3+4+5+6+7=28
-1+0+1+2+3+4+5+6+7+8=35...
Great! (There's a way to make this pattern work even without using negative numbers - can you spot it?) Why are all these patterns arising?
Becky spotted a different type of pattern:
We found out that powers of 2 (2, 4, 8, 16...) can never be made by adding together consecutive numbers together.
Interesting! I wonder why?
The Lumen Christi team give a way of constructing lots of multiples of odd numbers:
We worked out that if you divide a multiple of 3 by 3, and call the answer n, then your original number is the sum of (n-1), n and (n+1).
Then we discovered that the multiples of 5 can be written as 5 consecutive numbers. It's the same as the rule for 3 consecutive numbers. Take a number and divide it by 5, call it n, and then your number is the sum of (n-2), (n-1), n, (n+1) and (n+2).
We then made a conjecture that since it is true for 3 and 5, it would also work for 7, 9 and any other odd number. We tested it, and it worked. For example, 63 is a multiple of 7 and 9:
7 numbers: 6+7+8+9+10+11+12=63
(63/7 = 9)
9 numbers: 3+4+5+6+7+8+9+10+11=63
(63/9 = 7)
How could we take this investigation further? Arthur asked:
Are there any other patterns?
Can we explore the powers of two further?
Is there a nice way to write certain numbers (for example, every other even number) as a sum of consecutive numbers?
Ottilie suggested:
Instead of adding, you could multiply the consecutive numbers, and see what patterns come up. You could also only add consecutive even numbers, or only consecutive odd numbers. These things could all have something in common, or there could be a pattern between them, or nothing at all, maybe?
Magnus asked:
Is the rule that the powers of two can never be made always true? Can all numbers except the powers of two be made?
Great questions!
By the way, Abhi sent us a nice algebraic proof that powers of 2 can never be made:
Case 1: can we make $2^n$ from an odd number of consecutive numbers?
An odd number of consecutive numbers has a whole number as an average. This average is always the middle number. So, that means that the sum of the numbers will be:
Sum = average $\times$ number of consecutive numbers.
= whole number $\times$ odd number
This means the sum has an odd number as a factor. But $2^n$ cannot have an odd number as a factor. This proves that an odd number of consecutive numbers cannot add to make $2^n$.
Case 2: can we make $2^n$ from an even number of consecutive numbers?
An even number of consecutive numbers will not have a whole number as an average. The average will be the average of the two middle numbers. So:
Sum = (sum of two middle numbers) $\times \frac{1}{2} \times$ number of consecutive numbers
= (sum of two consecutive numbers) $\times$ ($\frac{1}{2}\times$ Even number)
= (sum of two consecutive numbers) $\times$ whole number
But if you add two consecutive numbers, the answer is always an odd number. So a sum like this must have an odd number as a factor again - but $2^n$ doesn't. This proves that an even number of consecutive numbers cannot add to make $2^n$.
Nicely done!
Teachers' Resources
Why do this problem?
This problem offers a simple context to begin exploration that naturally leads to some interesting conjectures about properties of numbers, and the possibility of developing some quite sophisticated algebraic arguments and proofs.
Possible approach
This printable worksheet may be useful: Summing Consecutive Numbers.
One approach is to introduce the lesson in the way it is introduced on the video, with students suggesting numbers and then the teacher at first, and then the whole class, finding ways to express them as consecutive sums.
Here is an alternative approach:
"Can someone give me a set of two or more consecutive numbers?"
Write a few sets on the board.
"What totals do we get by adding the consecutive numbers in these sets?"
Write + signs in between the lists of numbers.
"These totals are all examples of numbers that can be written as the sum of consecutive numbers. I wonder if all numbers can be written in this way ”¦"
"How about trying to write the numbers from $1$ to $30$ as sums of consecutive numbers?"
Give students time to work in pairs on filling in the gaps from $1$ to $30$. While they are working, write the numbers from $1$ to $30$ on the board ready to collect together the sums the class have found.
If students ask about negative numbers, one possible answer is: "Stick to positive numbers for now, and then perhaps investigate negative numbers later."
Once most pairs have filled in most of the gaps, collect their results on the board.
"Spend a minute looking at these results and then be prepared to talk about anything interesting that you notice."
Give them time to think on their own at first and then share ideas with their partner, before discussion with the whole class.
Next, collect together any noticings, and write them on the board in the form of questions or conjectures. If such conjectures are not forthcoming, here are some suggested lines of enquiry:
• "I wonder if we could write every number as the sum of consecutive numbers?"
• "Some numbers can be written in more than one way! I wonder which ones?"
• "9, 12 and 15 can all be written using three consecutive numbers. I wonder if all multiples of 3 can be written in this way?"
• "Maybe you could write the multiples of 4 if you used four consecutive numbers ..."
Allow pairs time to work on the conjectures of their choosing, reminding them that they will need to provide convincing arguments to explain any of their conclusions.
If appropriate, bring the class together to spend some time discussing algebraic representations of consecutive numbers ($n, n+1, n+2...$) to give students the tools to create algebraic proofs.
Finish the lesson by challenging students to express numbers that you have chosen as a sum of consecutive numbers in as many ways as possible - e.g. 45 can be expressed as the sum of consecutive numbers in 5 different ways.
Key questions
Possible support
9 = | 4+5 | 2+3+4 | ||
10 = | 1+2+3+4 | |||
11 = | 5+6 | |||
12 = | 3+4+5 | |||
13 = | 6+7 | |||
14 = | 2+3+4+5 | |||
15 = | 7+8 | 4+5+6 | 1+2+3+4+5 |
Possible extension
Challenge students to find an efficient way to calculate how many different ways $x$ can be expressed as a sum of consecutive numbers, for any $x$.
Marbles in a box
Problem
Marbles in a Box printable sheet - problem
Marbles in a Box printable sheet - methods
Imagine a three dimensional version of noughts and crosses where two players take it in turn to place different coloured marbles into a box.

The box is made from 27 transparent unit cubes arranged in a 3-by-3-by-3 array.
The object of the game is to complete as many winning lines of three marbles as possible.
How many different winning lines are there?
Once you have thought about how you might tackle the problem, click below to see four different methods for working out the number of winning lines in a $3 \times 3 \times 3$ cube.
Alison's method
- at a vertex
- at the middle of an edge
- in the centre of a face
From a vertex there are $7$ other vertices that you can join to in order to make a winning line. $7 \times 8 = 56$ lines, but this counts each line from both ends, so there are $28$ 'vertex' winning lines.
From the middle of an edge there are $3$ other middles-of-edges that you can join to in order to make a winning line. $3 \times 12 = 36$ lines, but this counts each line from both ends so there are $18$ 'middle of edge' winning lines.
From the centre of each face there is one winning line, joining to the opposite face, so there are $3$ 'centre of face' winning lines.
So in total, there are $28 + 18 + 3 = 49$ winning lines.
James' method
- Diagonal
- Not diagonal
There are $9$ from front to back.
There are $9$ from left to right.
There are $9$ from top to bottom.
Considering the diagonal winning lines:
On each layer there are $2$ diagonal winning lines so:
There are $6$ from front to back.
There are $6$ from top to bottom.
There are $6$ from left to right.
There are $4$ lines from a vertex to a diagonally opposite vertex.
In total, there are $27+18+4=49$ winning lines.
Caroline's method
- along an edge of the cube
- through the middle of a face
- through the centre of the cube
There are $12$ edges on a cube so there are $12$ winning lines along edges.
There are $6$ faces on a cube, and $4$ winning lines that pass through the middle of each face, so there are $24$ winning lines through the middle of faces.
Finally we need to consider the winning lines that go through the centre cube:
vertex to opposite vertex: $4$
middle of edge to middle of opposite edge: $6$
middle of face to middle of opposite face: $3$
In total, there are $12 + 24 + 4 + 6 + 3 = 49$ winning lines.
Grae's method
- in each horizontal plane
- in each vertical plane from left to right
- in each vertical plane from front to back
- in the diagonal planes
On a plane there are $8$ winning lines.
In the cube, there are $3$ horizontal planes, so $8 \times 3 = 24$ winning lines.
There are also $3$ vertical planes going from left to right, but now with only $5$ new winning lines per plane, as the $3$ horizontal lines have already been counted. So $5 \times 3 = 15$ winning lines.
On the $3$ vertical planes going from front to back, we now only have $2$ new (diagonal) winning lines per plane, as the $3$ horizontal and $3$ vertical lines, have already been counted. So $2 \times 3 = 6$ winning lines.
Finally, there are also diagonal planes to consider. There are $4$ winning lines going through the centre, from corner to diagonally opposite corner.
In total, there are $24 + 15 + 6 + 4 = 49$ winning lines.
Try to make sense of each method.
Now, try to adapt each method to work out the number of winning lines in a $4 \times 4 \times 4$ cube.
Can you adapt the methods to give a general formula for any size cube?
Check that each method gives you the same formula.
You may be interested in the other problems in our Reasoning Geometrically Feature.
Getting Started
Take a look at Painted Cube to think about how to visualise parts of a cube.
Student Solutions
Joel and Sarah from Sawston Village College, and Ethan from North Cross in New Zealand, found alternative methods for working out the number of different winning lines in a $3 \times 3 \times 3$ cube. Here is the description of Joel and Sarah's method. Ethan's method is shown below. Congratulations! Finding all the lines once, and none of them twice, is a real challenge.
Therefore, 8 regular solutions for a regular tic tac toe board x2 = 16.
24+16+9 = 49 winning lines.
The second part of the problem asked if it was possible to adapt the methods described for the $3 \times 3 \times 3$ cube to give a general formula for any size cube.
Jamal from King Edward VII School, Sheffield managed to adapt Caroline's method to a $4 \times 4 \times 4$ cube.
Remember that on a $3 \times 3 \times 3$ cube all winning lines must pass either:
- along an edge of the cube
- through the middle of a face
- through the centre of the cube
but these must be changed to make it work for a $4 \times 4 \times 4$ cube.
There are still 12 edges on a $4 \times 4 \times 4$ cube so there are 12 winning lines along edges.
Since on the larger cube there is no 'middle' of a face, I counted all the lines on each face that aren't along the edge instead, each one passes through the 'middle' four pieces of a face. There are 2 diagonals, 2 horizontal and 2 vertical lines on each of the 6 faces, so that's 36 more lines.
Again the $4 \times 4 \times 4$ doesn't have a 'centre' piece so I counted all the lines through the central $2 \times 2 \times 2$ block. I found there is exactly one winning line through any two of those eight pieces in the block. There are (8x7)/2 =28 pairs because I can choose from eight pieces for the first one, then from the remaining seven for the second, but I divide by two because it
doesn't matter in what order I choose at the pair.
Adding these up I have 12 + 36 + 28 = 76 winning lines.
Niharika used similar reasoning to find the number of winning lines in an $n \times n \times n$ cube.
Rachael from Lancaster Girls' Grammar School used James' method to find the same result, and then generalised the answer to $n \times n \times n$ cubes.
Considering the non-diagonal winning lines first:
There are 16 from front to back.
There are 16 from left to right.
There are 16 from top to bottom.
Considering the diagonal winning lines:
On each layer there are 2 diagonal winning lines so:
There are 8 from front to back.
There are 8 from top to bottom.
There are 8 from left to right.
There are 4 lines from a vertex to a diagonally opposite vertex.
In total, there are 48+24+4 = 76 winning lines.
If I now take a cube of size nxnxn, there are n2 non-diagonal lines from front to back, and left to right, and top to bottom. That makes 3n2 lines. Counting the diagonal ones, there are 2 in each of the n layers, in each of the 3 dimensions; so 6n lines. Then there's the four lines from a vertex to a diagonally opposite vertex. In total there are 3n2 + 6n +4
lines.
Llewellyn from Cowbridge Comprehensive School saw that Alison's method generalises easily, jumping straight to the nxnxn formula.
A cube has 8 vertices, 12 edges and 6 faces. Correspondingly there are three kinds of pieces: vertex, edge, and face. An $n \times n \times n$ cube has 8 vertex peices, 12(n-2) edge pieces and 6(n-2)2 face pieces.
From a vertex piece there are 7 other vertex pieces that you can join to in order to make a winning line. 7×8 = 56 lines, but this counts each line from both ends, so there are 28 vertex winning lines.
From an edge piece there are 3 other edge pieces that you can join to in order to make a winning line. Remembering that this counts each line from both ends there are 3×12(n-2)/2 = 18n - 36 edge winning lines.
From a face piece there is one winning line, joining to the opposite face, so there are 6(n-2)2/2 = 3n2 - 12n + 12 face winning lines.
So in total, there are 28 + 18n - 36 + 3n2 - 12n + 12 = 3n2 + 6n + 4 winning lines. That means there are 76 winning lines in a 4x4x4 cube and 364 winning lines in a 10x10x10 cube.
Leah from St Paul's Girls' School saw how to adapt Grae's method to a solution for 4x4x4 cubes.
The winning lines may be counted by looking at lines:
- in each horizontal plane
- in each vertical plane from left to right
- in each vertical plane from front to back
- in the diagonal planes
On a non-diagonal plane there are 10 winning lines (4 horizontal, 4 vertical and 2 diagonal lines).
In the cube, there are 4 horizontal planes, so 10×4=40 winning lines.
There are also 4 vertical planes going from left to right, but now with only 6 new winning lines per plane, as the 4 horizontal lines have already been counted. So 6×4=24 winning lines.
On the 4 vertical planes going from front to back, we now only have 2 new (diagonal) winning lines per plane. So 2×4 = 8 winning lines.
Finally, there are also diagonal planes to consider. There are 4 winning lines going from corner to diagonally opposite corner.
In total, there are 40 + 24 + 8 + 4 = 76 winning lines.
It is possible to adapt Grae's and Caroline's methods to the general formula and you might like to try using Leah's or Jamal's work to help you get started. Of course it's easier now you know what formula you're looking for!
Teachers' Resources
Why do this problem?
As well as giving students an opportunity to visualise 3-D solids, this problem provokes the need for students to work systematically. Counting the winning lines in an ad hoc way will result in double-counting or missed lines, with students getting many different answers. It is only by working in a systematic way that students can convince themselves that their answer is correct. By offering a variety of methods, we hope students will evaluate the merits of the different approaches, and recognise the power of methods which make it possible to generalise.
Possible approach
These printable worksheets may be useful:
Marbles in a Box
Marbles in a Box - Methods
"If I played a game of noughts and crosses, there are eight different ways I could make a winning line. I wonder how many different ways I could make a winning line in a game of three-dimensional noughts and crosses?"
The image from the problem could be used to show one example of a winning line.
Give students time to discuss with their partners and work out their answers. While they are working, circulate and observe the different approaches that students are using, and challenge them to explain any dubious reasoning. After a while, stop the group to share their results, perhaps writing up all their answers on the board (it is likely that there will be disagreement!).
"It's often difficult to know we have the right answer to a problem like this, because there is a danger of missing some lines or counting some lines twice. Here are the systematic methods that four people used to work out the number of winning lines. For each method, try to make sense of it, and then adapt it to work out the number of
winning lines of 4 marbles in a 4 by 4 by 4 cube."
The methods are arranged two to a sheet, so you could give each half of the class a different pair of methods to work on, or alternatively you could give everyone all four methods.
"Once you have adapted the methods for a 4 by 4 by 4 cube, have a go at working out what would happen with some larger cubes, and perhaps try to write down algebraically how many lines of n marbles there would be for an n by n by n cube."
Bring the class together and invite students to present their thinking, by asking them to explain how to work out the number of winning lines in a 10 by 10 by 10 version of the game.
Finally, work together on creating formulas using each method for the number of winning lines in an n by n by n game (or gather together on the board the algebraic expressions they found earlier) and verify that they are equivalent.
Key questions
How can you categorise the types of winning line, to make sure you don't miss any?
How would you extend Alison's/James'/Caroline's/Grae's method to count the number of winning lines in a 4 by 4 by 4 cube? Or an n by n by n cube?
Possible support
Painted Cube offers students the opportunity to work with the structure of a cube and consider faces, edges and vertices.
Possible extension
Extend the cubic 'grid' to a cuboid, possibly 4 by 3 by 3 to start with, and ultimately $n$ by $m$ by $p$, always looking for lines of 3 - unless students want to look for other length lines (they could look for lines of 2 on the 3 by 3 by 3 grid).
Route to infinity
Problem
Route To Infinity printable sheet
Take some time to look at the route followed by the arrows in this diagram.
Then look away and describe the path to a friend.
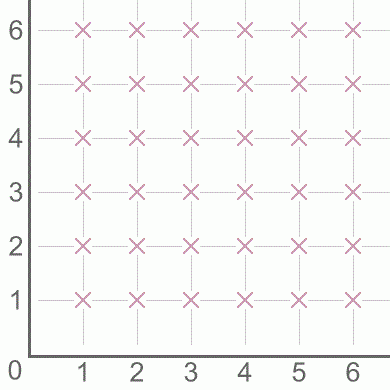
If the pattern of arrows continues for ever, which point will the route visit immediately after (18,17)? Explain how you know.
How many points will be visited before the route reaches the point (9,4)?
Explain how you worked it out.
Which point will be the $1000^{th}$ to be visited?
Click here for a poster of this problem.
Getting Started
Take a look at the co-ordinates of the points along the route.
What do you notice about the order in which the route visits the points?
When do the arrows point up, and when do they point down?
Student Solutions
After (18, 17), the route will visit (19, 16). Lots of people got this right - let's have a look at just a few of their solutions:
Tiberiu noticed:
I see that the route goes upwards through the points with coordinates that are equal (eg. 18,18) and downwards through the points with coordinates that are adjacent (eg. 18,17). Therefore the next coordinate is (19,16).
Lawrence, from Chircher's College, worked it out like this:
Looking at the diagram you can tell that if the x coordinate of the bottom right point on a line is even, the arrows in the line go down and right, otherwise, they go up and left. The point (18,17) is on the line with bottom right point (18+17-1,1) = (34,1). Because 34 is even, the arrows go down and right, so the next point is (19,16).
Alex, from Dixons City Academy, spotted the same thing, in a different way:
The numbers all lie on lines with equations x+y=n, where n is some integer. If n is odd, then the line begins at (1, n-1). These arrows point towards the bottom right. If n is even, the line begins at coordinate (n-1, 1) and the direction of the line is towards the top left. Notice that 18+17=35, so the coordinate (18, 17) is on the line x+y=35.
Segev, Adam, Yoav, Daniel and Jonathan from JFS answered the second part of our question:
There are 74 points visited before the route reaches the point (9,4). The number of points visited follows the triangle numbers.
Number of points visited up to (1,1): 1
Number of points visited up to (2,1): 3
Number of points visited up to (1,3): 6
Number of points visited up to (4,1): 10
etc.
Therefore the number of points visited up to (1,11) is 66.
It is worth noticing here that because $9+4=13$ is odd, the line points towards the top left, so we are starting at (1,11).
Then, we need to visit 8 points on the diagonal before reaching (9,4), which are to (1,12), (2,11), (3,10), (4,9), (5,8), (6,7), (7,6) and (8,5) before reaching (9,4).
This is a total of $66+8=74$ points visited before (9,4).
The diagram below shows the 66 steps in blue, and then these final steps in red. Although there are nine red steps marked, only eight are counted as it is the number before (9,4) that is requested.
There were far too many great solutions to list here - thanks to everyone who submitted one!
Teachers' Resources
Why do this problem?
This problem offers a good opportunity for students to discuss images and find convincing arguments for their solutions.
Reuben Hersh has written that:
"In the classroom, convincing is no problem. Students are too easily convinced. Two special cases will do it."
This problem offers an opportunity to ensure that students are justified in generalising from the particular cases that they have selected.
Possible approach
This printable worksheet may be useful: Route To Infinity
You may be interested in our collection Dotty Grids - an Opportunity for Exploration, which offers a variety of starting points that can lead to geometric insights.
Show the diagram. It's available dynamically on a PowerPoint slide, or you could use the poster for a static version of the image.
Possible support
Before working on this problem, spend time on Cops and Robbers and Coordinate Patterns to develop students' fluency with coordinates.
Possible extension
What's Possible?
Problem
What's Possible? printable worksheet
You may be interested in Hollow Squares which offers an alternative way of thinking about the same underlying mathematics.
Many numbers can be expressed as the difference of two perfect squares. For example, $$20 = 6^2 - 4^2$$ $$21 = 5^2 - 2^2$$ $$36 = 6^2-0^2$$
How many of the numbers from $1$ to $30$ can you express as the difference of two perfect squares?
Here are some questions to consider:
What do you notice about the difference between squares of consecutive numbers?
What about the difference between the squares of numbers which differ by $2$? By $3$? By $4$...?
When is the difference between two square numbers odd?
And when is it even?
What do you notice about the numbers you CANNOT express as the difference of two perfect squares?
You may want to take a look at Plus Minus next.
Getting Started

Student Solutions
Hannah from Munich International School worked out which numbers between 1 and 30 were possible:
1: $1^2 - 0^2$ is $1 - 0 = 1$
2: does not work
3: $2^2 - 1^2$ is $4 - 1 = 3$
4: $2^2 - 0^2$ is $4 - 0 = 4$
5: $3^2 - 2^2$ is $9 - 4 = 5$
6: does not work
7: $4^2 - 3^2$ is $16 - 9 = 7$
8: $3^2 - 1^2$ is $9 - 1 = 8$
9: $5^2 - 4^2$ is $25 - 16 = 9$
10: does not work
11: $6^2 - 5^2$ is $36 - 25 = 11$
12: $4^2 - 2^2$ is $16 - 4 = 12$
13: $7^2 - 6^2$ is $49 - 36 = 13$
14: does not work
15: $8^2 - 7^2$ is $64 - 49 = 15$
16: $5^2 - 3^2$ is $25 - 9 = 16$
17: $9^2 - 8^2$ is $81 - 64 = 17$
18: does not work
19: $10^2 - 9^2$ is $100 - 81 = 19$
20: $6^2 - 4^2$ is $36 - 16 = 20$
21: $11^2 - 10^2$ is $121-100 = 21$
22: does not work
23: $12^2 - 11^2$ is $144-121=23$
24: $7^2-5^2$ is $49 - 25 = 24$
25: $13^2 - 12^2$ is $169-144=25$
26: does not work
27: $14^2 - 13^2$ is $196 - 169 = 27$
28: $8^2 - 6^2$ is $64-36 = 28$
29: $15^2 - 14^2$ is $225 - 196 = 29$
30: does not work
You can express 22 of the 30 numbers as a difference of two perfect squares. A pattern occuring throughout these solutions is that all odd numbers can be represented by a difference of two perfect squares, as well as all numbers resulting in an integer when divided by four.
Joshua from St John's School used algebra to show how odd numbers and multiples of four could be made:
You can make every odd number by taking consecutive squares.
$(n+1)^2 - n^2 = 2n+1$, every odd number can be written in the form $2n+1$.
Similarly, you can make every multiple of 4 by taking squares with a difference of 2.
All other numbers you can't make:
$(n+x)^2 - n^2 = x^2 + 2nx = x(x+2n)$
If $x$ is odd then $x^2$ is also odd and $2nx$ is even. An odd plus an even is odd.
If $x$ is even then $x^2$ is a multiple of $4$ and $2nx$ is also a multiple of $4$ so $(n+x)^2 - n^2$ is a multiple of $4$.
Numbers such as 2,6,10 etc can't be made because these are made by multiplying an even by an odd.
Patrick from Woodbridge School and Richard from Mearns Castle School both used a formula for the difference of two squares to investigate which numbers were possible. Here is Patrick's solution:
$a^2 - b^2= (a + b)(a - b)$
Thus, a number $n$ can only be a difference of two squares if it has two factors of the form $(a + b)$ and $(a - b)$, where $a + b \geq \sqrt{n}$ and $a - b \leq \sqrt{n}$.
If $1$ could be written as the difference of squares then $(a + b) = (a - b) = 1$, as the only factor of $1$ is $1$.
Thus, $a = 1$ and $b = 0$. So $1 = 1^2 - 0^2$.
If $2$ could be written as the difference of two squares then $(a + b) = 2$ and $(a - b) = 1$, as $2$ is prime, and clearly $a - b < a + b$ for positive $b$.
Thus, solving the simultaneous equations, $b = 2 - a$, so $a - (2 - a) = 1$, $2a = 3$ and $a = \frac{3}{2}$. Thus, $2$ is not representable as the difference of two integer squares.
This method can be generalised for any prime $p$ by solving $(a + b) = p$ and $(a - b) = 1$
Adding the equations gives $2a = p + 1$, so $a = \frac{p+1}{2}$. Since $a - b = 1$, $b = a-1$, so $b = \frac{p+1}{2} - 1 = \frac{p-1}{2}$. Thus, any odd prime can be written as the difference of two squares.
Any square number $n$ can also be written as the difference of two squares, by taking $a = \sqrt{n}$ and $b = 0$.
Generally, a number can be written as the difference of two squares if it has two factors of the same parity, since if $a + b$ is odd and $a - b$ is even, when the two equations are added we would get $2a$ odd, so the solution would not be an integer. So a number cannot be written as a difference of two squares if and only if it is equivalent to $2$ mod $4$ (leaves a remainder of $2$ when divided by $4$).
Richard from Comberton Village College considered prime factorisation:
An integer $x$ can be written as the difference between two square integers unless it contains only one $2$ in its prime power factorisation.
Proof: $x=a^2 - b^2$ can be rewritten in the form $(a+b)(a-b)$.
Suppose $u$ and $v$ are two factors of $x$ such that $u = a + b$ and $v = a - b$.
Subtracting the two equations, we get $u - v = 2b$.
This tells us that the difference between the two factors will always be even if the number can be written in this form.
Now, to get an even difference, we either need $u$ and $v$ to be both odd, or both even. Therefore, any number with either two even or odd factors can be written in this form.
This leaves numbers which will always have one odd and one even factor.
If $v$ is an odd factor of $x$, it must have a prime factorisation of only odd numbers.
Suppose $u$ is an even factor of $x$ and contains more than one $2$ in its prime factorisation.
This means that $u$ could be divided by two and still be even, and at the same time $v$ could be doubled so that it too is even. This means that $x$ has a factor pair which are both even, and so can be written as the difference of two squares.
If both factors are odd an even difference will also be attained, so if there are no twos in the prime factorisation of $x$, then x can be written as the difference of two squares.
This leaves the case where there is exactly one $2$ in the prime factorisation. This means that one factor must contain the $2$ and not the other, and that the factors cannot be manipulated in the fashion described before. This will always leave factors with an odd difference suggesting $x$ cannot be written as the difference of two squares.
This also gives a very nice algorithm for finding two squares which have a difference of $x$:
1. Establish how many $2$s are in the prime factorisation of $x$. If there is only one, x cannot be written as the difference of two squares.
2. Choose a factor pair which are both even or both odd, call them $u$ and $v$. Find their difference and divide it by 2 to find the smaller square. $\frac{u - v}{2}=b$ from before.
3. Square $b$ and add it to $x$. Square root the sum and that is $a$.
We now have $x = a^2 - b^2$.
For example, $60 = 2 \times 2 \times 3 \times 5$. There is more than one 2 so it is possible.
The two factors $2 \times 5 = 10$ and $2 \times 3 = 6$ give a difference of 4. Halve it to get $b = 2$.
$x + b^2 = 60 + 4 = 64$. $\sqrt{64} = 8$, so $a = 8$.
This gives us $60 = 8^2 - 2^2$.
We could also have taken $u = 2 \times 3 \times 5$ and $v = 2$, which gives a difference of 28. Halve it to get $b=14$.
$x + b^2 = 60 + 196 = 256$.
$\sqrt{256} = 16$ so $a = 16$.
$16^2 - 14^2 = 60$
Teachers' Resources
Why do this problem?
This problem starts by asking students to find which numbers can be expressed as the difference of two square numbers, and then suggests some possible avenues for exploration. This can then be used as a springboard to generalisations and the use of algebra for justifications and proof. Along the way, students have the opportunity to make use of the
important identity $a^2 - b^2 = (a + b)(a - b)$.
An alternative to this problem which some students may find more accessible is Hollow Squares.
Possible approach
This printable resource may be useful: What's Possible? worksheet.You may wish to introduce the problem like this:
Ask students for a two-digit number under 30. Write it on the board and express it as the difference between two squares. Repeat several times.
Challenge students to find all numbers between 1 and 30 that can be expressed as the difference of two squares. Encourage them to find more than one solution where possible.
Once students have had time to find most of the answers, ask them to share what they found and list their answers on the board.
'Have a look at the results so far. What do you notice?'
Give students a couple of minutes to talk to their partners before bringing the class back together.
Here are some of the conjectures and questions that may emerge:
• The difference between squares of consecutive numbers is equal to the sum of the consecutive numbers.
• What happens to the difference when I square two numbers that differ by 2?
• Numbers that can be made in more than one way have lots of factors.
• When is the difference between two square numbers odd?
• And when is it even?
• Why are some numbers impossible to make?
• Can we predict which numbers are impossible to make?
Alternatively, you could introduce the problem like this:
Bring the class back together and invite those students who have useful insights to share them with the class. You may wish to introduce an algebraic and a geometric approach to proving one particular conjecture: for example, that the difference between consecutive squares is always odd. A diagrammatic method for calculating the difference of two squares is explored in the problem Plus Minus.
Now give the class some time to work on proving their conjectures.
You may also wish to set the following challenge:
"In a while, I'm going to give you a number and ask you to quickly find one or more ways to write it as the difference of two squares, or to convince me that it can't be done. Can you develop a strategy that will help you do this?"
Your plenary could involve students presenting their findings to the rest of the class. Expect students to be clear and rigorous in their justifications. Encourage them to challenge any proofs that lack clarity and rigour, and suggest ways of improving them.
Key questions
Possible extension
Possible support
1 | 2 | 3 | |
1 | $1^2-0^2$ | ||
2 | |||
3 | $2^2-1^2$ | ||
4 | $2^2-0^2$ | ||
5 | $3^2-2^2$ | ||
6 | |||
7 | $4^2-3^2$ | ||
8 | $3^2-1^2$ | ||
9 | $5^2-4^2$ | $3^2-0^2$ |
Painted Cube
Problem
Painted Cube printable worksheet
Imagine a large cube made up from $27$ small red cubes.
Imagine dipping the large cube into a pot of yellow paint so the whole outer surface is covered, and then breaking the cube up into its small cubes.
How many of the small cubes will have yellow paint on their faces?
Will they all look the same?
Now imagine doing the same with other cubes made up from small red cubes.
What can you say about the number of small cubes with yellow paint on?
Click here for a poster of this problem.
Getting Started
How many vertices, faces and edges are there in a cube?
Where are the cubes with no faces painted?
Where are the cubes with 1, 2, 3, 4, 5, 6 faces painted?
Student Solutions
We received many correct solutions to this problem - from Adam from Knoxdale P. S., Ramsudheer from Smith Elementary School, Ojaswi, Bill from King's St. Alban's, Chantell, Billy, Connor and Chanel from Arunside, Rajeev from Fair Field Junior School, Jessica from Beeston Rylands Junior School, Finlay from Gledhow Primary School, Olivia and Martha from St.Johns C.E. Primary School, Yuki from King's School Ely, Belky, Mark from Gledhow Primary School, James and Arjun from Wilson's, William from Barnton Community Primary School Alice, Joanna from Woodmill High School, Derek, Robert from Ardingly College Junior School, Joe from Lady Manners School in Bakewell and students from Rawdon Littlemoor Primary School. Well done to you all.
Alan wrote:
Chantell, Billy, Connor and Chanel sent us this clear table summarising their findings. Rajeev sent us a very similar table of results.
Yuki noticed that:
Students from Crestwood College also mentioned this.
Arjun also worked on this:
Mark from Gledhow Primary School produced this spreadsheet.
Robert summarised his findings as follows:
"Let n= number to be cubed
No. of small cubes with 6 red faces = (n-2)$^3$
This is because the cubes in the centre remain clean, so you must take one off either edge and then cube it.
No. of small cubes with 5 red faces = 6(n-2)$^2$
This is because there are 6 faces to the cube, and only the ones not on the edge remain clean on 5 sides. So you must take one off either edge, then square it, then multiply it by the 6 faces.
No. of small cubes with 4 red faces = 12(n-2)
This is because there are 12 non-corner edges which is multiplied by n (which = 1 whole edge) -2 for the 2 corners.
No. of small cubes with 3 red faces = 8
This is because there are always 8 corners
The total No. of small cubes is always n cubed"
Alice described her findings in a similar way:
"First of all I imagined a 3x3x3 cube being dipped in paint - that's how I worked the first one out then we worked out that
1) the number of cubes with 6 red faces equalled (n-2)$^3$, like when you take the skin off a square orange, taking a layer off each side
2) 5 red faces 6(n-2)$^2$, which is like the above but for the faces instead of the middle (it's squared not cubed ) and you have to multiply it by 6 because there are 6 faces
3) 4 red faces 12(n-2) this is the edges, take 2 for the corners and multiply it by 12 because that's how many edges there are
4) 3 red faces always got to be eight because these are corners (unless your cube is 1x1x1)
5) total number of small cubes is n$^3$
Joe, Derek and Alice completed the table of results:
Size of large cube |
No. of small cubes with 6 red faces |
No. of small cubes with 5 red faces |
No. of small cubes with 4 red faces |
No. of small cubes with 3 red faces |
Total No. of small cubes |
3 x 3 x 3 |
1 |
6 |
12 |
8 |
27 |
4 x 4 x 4 |
8 |
24 |
24 |
8 |
64 |
5 x 5 x 5 |
27 |
54 |
36 |
8 |
125 |
6 x 6 x 6 |
64 |
96 |
48 |
8 |
216 |
|
|
|
|
|
|
|
|
|
|
|
|
10 x 10 x 10 |
512 |
384 |
96 |
8 |
1000 |
23 x 23 x 23 |
9261 |
2646 |
252 |
8 |
12167 |
n by n by n |
(n-2)$^3$ |
6(n-2)$^2$ |
12(n-2) |
8 |
n$^3$ |
NB. The values for n are correct unless n = 1.
When n = 1 the single cube will have no red faces.
In her conclusion Alice added that in the second column the numbers were all cubic numbers, in the third column square numbers times 6 and in the fourth column multiples of 12.
Belky explained how she reached her results. This is how she arrived at a formula for the number of cubes with two faces painted :
Both William and Derek showed that:
We can verify that all cubes have been accounted as:$$(n-2)^3 + 6(n-2)^2 + 12(n-2) + 8 $$ $$= (n-2) (n^2 - 4n + 4) + 6 (n^2 - 4n + 4) + 12n - 24 + 8 $$ $$ = n^3 - 4n^2 + 4n - 2n^2 + 8n -8 + 6n^2 - 24n + 24 + 12n - 24 + 8 $$ $$ = n^3 $$
Derek added this diagram to support his conclusions:
Mollie and Hannah from Comberton Village College also sent in some excellent solutions; follow the links below to see their work:
Mollie's solution
Hannah's solution
Teachers' Resources
Why do this problem?
Exploring a variety of painted cubes may produce patterns which students can describe spatially, numerically and algebraically. Students can appreciate the benefits of keeping a clear record of results, and apply their insights from the first case to ask themselves questions about further cases.
This problem lends itself to collaborative working, both for students who are inexperienced at working in a group and students who are used to working in this way.
Many NRICH tasks have been designed with group work in mind. Here we have gathered together a collection of short articles that outline the merits of collaborative work, together with examples of teachers' classroom practice.
Possible approach
This printable worksheet may be useful: Painted Cube.
This approach could be used if you want the focus to be on the mathematical structure of the problem:
"Here is a 3 by 3 by 3 cube made up of 27 smaller cubes. Imagine I dipped it in a pot of yellow paint so that each face of the large cube was covered. Then after the paint has dried, imagine I split it into the 27 original small cubes. Can you work out how many cubes will have no paint on them? How many will have just one face painted? Or two faces painted, and so on."
Give students time to discuss with their partners and work out their answers. While they are working, circulate and observe the different approaches that students are using, and challenge them to explain any dubious reasoning.
Bring the group together to share their responses. Collect the answers for the number of cubes with 0, 1, 2, 3... faces painted, and note that they add up to 27. Invite students to explain how they worked it out.
"I'd like you to work on some cubes of different sizes until you are confident that you can always work out how many cubes will have 0, 1, 2 and 3 faces painted. In a while, I'll be choosing a much larger cube at random, and you?ll need to have an efficient method of working it out."
Here are some prompts that could be used if students get stuck:
"Where are the cubes with no faces painted?"
"Where are the cubes with 1, 2, 3 faces painted?"
"How many of each type of cube would you have in a 4 by 4 by 4? in a 7 by 7 by 7? in an n by n by n cube?"
Bring the class together and challenge them to explain how they can work out the number of cubes of each type in a 10 by 10 by 10 painted cube. Depending on the students' experience of working with algebra, you could work together on creating formulas for the number of cubes of each type in an n by n by n cube. The Solution contains a table that shows the results very clearly.
This approach could be used if you want the focus to be on developing groupwork:
This is an ideal problem for students to tackle in groups of four. Allocating these clear roles (Word, pdf) can help the group to work in a purposeful way - success on this task should be measured by how effectively the group work together as well as by the solutions they reach.
Introduce the four group roles to the class. It may be appropriate, if this is the first time the class have worked in this way, to allocate particular roles to particular students. If the class work in roles over a series of lessons, it is desirable to make sure everyone experiences each role over time. For suggestions of team-building maths tasks for use with classes unfamiliar with group work, take a look at this article and the accompanying resources.
Hand out this task sheet (Word, pdf) to each group, and make it clear that everyone needs to be ready to share their findings with the rest of the class at the end of the sessions. Exploring the full potential of this task is likely to take more than one
lesson, with time in each lesson for students to feed back ideas and share their thoughts and questions.
You may want to make isometric paper, cubes, poster paper, and coloured pens available for the Resource Manager in each group to collect.
While groups are working, label each table with a number or letter on a post-it note, and divide the board up with the groups as headings. Listen in on what groups are saying, and use the board to jot down comments and feedback to the students about the way they are working together.
You may choose to focus on the way the students are co-operating:
Group A - Good to see you sharing different ways of thinking about the problem.
Group B - I like the way you are keeping a record of people's ideas and results.
Group C - Resource manager - is there anything your team needs?
Alternatively, your focus for feedback might be mathematical:
Group A - I like the way you are considering the structure of the cube.
Group B - You've identified which cubes end up with one face painted - can you think of a way of quickly counting them?
Group C - Good to see that someone's checking that the answers are in line with your predictions.
Make sure that while groups are working they are reminded of the need to be ready to present their findings at the end, and that all are aware of how long they have left.
We assume that each group will record their diagrams, reasoning and generalisations on a large flipchart sheet in preparation for reporting back. There are many ways that groups can report back. Here are just a few suggestions:
- Every group is given a couple of minutes to report back to the whole class. Students can seek clarification and ask questions. After each presentation, students are invited to offer positive feedback. Finally, students can suggest how the group could have improved their work on the task.
- Everyone's posters are put on display at the front of the room, but only a couple of groups are selected to report back to the whole class. Feedback and suggestions can be given in the same way as above. Additionally, students from the groups which don't present can be invited to share at the end anything they did differently.
- Two people from each group move to join an adjacent group. The two "hosts" explain their findings to the two "visitors". The "visitors" act as critical friends, requiring clear mathematical explanations and justifications. The "visitors" then comment on anything they did differently in their own group.
Key questions
If your focus is mathematical, these prompts might be useful:
Where are the cubes with no faces painted?
Where are the cubes with 1, 2, 3, 4, 5, 6 faces painted?
How many of each cube will you have in an n by n by n cube?
How do your algebraic expressions relate to the geometry of the situation?
Possible support
By working in groups with clearly assigned roles we are encouraging students to take responsibility for ensuring that everyone understands before the group moves on.
Possible extension
Partly Painted Cube provides a suitable follow-up activity.
Amazing Card Trick
Problem
Amongst our trips was a second visit to the area around the island of Kekova (Turkey's largest, but quite small, island). The boat trip interspersed swimming and snorkelling with more intellectual activities including a look at the sunken city, views of Lycian tombs, and a trip to the medieval castle at Kaleucagiz. Last but not least, a sharing of card tricks with our tour guide Mehmet. My daughter and I showed Mehmet the Best Card Trick and in exchange he shared the following (amazing card trick) with us. Mehmet not only made the day memorable and enjoyable but left me with the problem of how the trick worked to solve. The mathematics is easy so I hope you will try to get to grips with it...........
Give a full deck of cards to someone in the audience and ask them to shuffle and cut them.
Take the pack face down and count out the first half of the pack, turning them face up onto a pile in front of the member of the audience.
When you have done this - pick up the 26 cards and place them face down back at the bottom of the pile you have in your hand.
Take three cards from the top of the pack and place them face up on the table. Then add enough cards to each (all face down) to make a total of 10.
So, if you turn up the 3
The three cards showing (face up) on the table are the 3
You should now be able to predict the 21st card down the rest of the pack sitting in your hand .
"And the 21st card will be..."
How is it possible to predict this card no matter what the three cards you turn over are?
Watch Charlie and Alison performing the Amazing Card Trick:
Getting Started
Do you need to use any of the 26 cards that you turned up at the start of the trick?
Is this always the case?
Student Solutions
Felix, Matthew, Alice, Robert, Hayden, Jenna, Catherine, James, James, Nick, Kieran, Kayleigh, Bethany, Luke and Matthew, all from Cupernham School sent in explanations which involved a similar argument. Correct explanations were also recieved from Andrei of School 205 Bucharest, Sophia of the Maths Club at Stamford High School and Matthew of Finley Middle School. Here is an explanation based on all of yours.
This card trick has nothing to do with magic, just mathematical thinking. When you do this trick, the total amount of cards on the table is 33.
It works because there are 3 cards you put down in the first place, the cards you add to them to make 10 and the number of cards down the pack. These make a total of 33 if you add them together. The number you memorise is the 7th card into the original half of 26. That is the top 26+7 into the original half equal 33 so that is how it works.
eg. If you lay down the 9 diamonds, 3 hearts and 6 clubs, it would look like this:
9 3 6 (three cards on the table)
You then lay down 1, 7 and 4 cards respectively (making a total of a12 +3 = 15 cards).
1 7 4
You then count down the pack 9+3+6 cards = 18 cards.
That is 15 cards on the table plus 18 cards down the pack- making 33.
9 3 6
-----
1 7 4
9 3 6
The 2 last rows show the cards that make up the original card to 10. These cards add up to 30. The top row is the original three cards, so if you add them on, the final total is 33. This is always true, because the three cards are made up to ten every time. This is because you have to make up the numbers on the cards, then deal out the original number again when you have made the prediction. The trick is when you deal out the 26 cards at the beginning, you take note of the 7th card. This is the card you predict.
If you do this trick properly it can be very entertaining!
Teachers' Resources
Here is a silent video with written instructions for each phase of the trick. It can be displayed on a loop while students are figuring out the trick, to remind them of the instructions if they forget.